XXXXXXXXXXXXXXXXXXXXXXXXXXXXXXXXXXXXXXXXXXXXXXXXXXXXXXXXXXXXXXXXXXXXXXXXXXXXXXXXXXXXXXXXXXXXXXXXXXXXXXXXXXXXXXXXXXXXXXXXXXXXXXXXXXXXXXXXXXXXXXXXXXXXXXXX''"> Glossary Terms
Note: | The terms and definitions in this appendix may have multiple meanings to different people. The definitions give here are as used in the context of this guide. |
Amplitude
Amplitude is half of the range of a cycle. It is the maximum less the minimum divided by two.
August Woehler
This German gentleman is probably the most famous of all fatigue researchers being the “Father of Fatigue” as many know him. He is responsible for the invention of the “stress-life” or “S-N” method of fatigue life prediction. See
Stress-Life (S-N), 540.
β-Solution
Biaxial - Biaxiality Ratio
For surface resolved stresses the two major principal stresses lie in the plane of the surface with the third principal stress being zero (normal to the surface). The principal stresses therefore, correspond to the X, Y, and Z=0 component stresses. The ratio of the minimum in-plane stress divided by the maximum in-plane stress defines the biaxiality ratio. This ratio can take on any number between -1 and 1. Zero indicates a uniaxial state of stress with only one principal stress being non-zero.
Broad Band
Compliance Function
For crack growth analysis, a compliance function needs to be defined. This is also known as a β-function or a K-solution. It is defined based on the crack geometry and the dimension of the specimen. In physical terms it is simply a measure of how the compliance (stiffness or flexibility) of the structure changes as the crack propagates. These functions take on the form of look up tables in the software and can be defined in terms of a polynomial equation or selected from a standard library set. They are then used in the crack growth rate (Paris) equation to determine the stress intensity for any given stress cycle and, in turn, used to determine a incremental crack size.
Component S-N
This is an S-N curve which is tied to a specific component geometry and relates nominal stress (S) to life. The stress that is looked up on the S-N curve is not the actual stress at the failure location (in general) but the stress as measured in a location away from the failure. This is usually because of the impracticality of placing a strain gauge at the failure location. The S-N curve can only be used for components with the same geometry (and material) as that used to create the S-N curve. The components themselves are used as test specimens to create the S-N curves (such as a weld class). These types of S-N curves are not used to identify the location of a possible fatigue failure since this location is already identified by the nature of the S-N curve. Instead they are used to evaluate resistance to variation of the loading. These S-N curves are generally used when the failure mechanism is not well modeled with material S-N curves or other methods. They represent a more macro way of characterizing the failure mechanism by building into the curve all influences on the fatigue life, i.e., plasticity, geometry, residual stress, etc. The challenge when using these S-N curves with FE analysis is to know where the measured or reference location is, which is the stress that should be used to look up damage using these S-N curves.
Crack Initiation
Crack Growth (Propagation)
Critical Plane Analysis
The principal stress tensor axis can rotate from time step to time step when subject to multiple load inputs. A fatigue analysis done at various angles is said to be a critical plane analysis. The plane that exhibits the most damage is said to be the critical plane. These types of analyses are typically illustrated using a polar plot of damage versus angle.
Cycles (cycle counting)
A stress or strain cycle is one event that may or may not cause damage. A single constant amplitude, fully reversed sine or triangle wave that passes upwards positively through its mean will register a stress cycle each time. Many methods of counting cycles in a randomly varying signal have been adopted and abandoned over the years. The rainflow cycle counting method is the most widely accepted. Another way to understand cycle counting is illustrated by the diagram where a portion of a time signal is stood on end and then each point is mapped onto stress-strain space. This simulates loading and unloading where the material may yield producing a hysteresis loop. Each loop represents one stress cycle. Cycles with noticeable inner area are damaging and cycles which appear as straight lines are purely elastic and cause no damage. For any time varying load, all cycles will fall with in one outer, large cycle representative of the maximum and minimum of the signal. So in short, cycle counting counts the number of hysteresis loops and keeps track of their range/mean or maximum/minimum values.
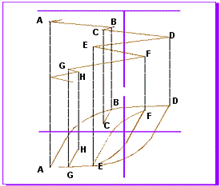
Cyclic Properties
Cyclic material properties are those that are obtained from a material test similar to a monotonic test with one big difference. The loading is reversed and cycled at various load levels. From these tests are derived the cyclic stress-strain curve and the strain-life curve used in crack initiation analysis. At each load level the stabilized hysteresis loop is extracted, its maximum stress/strain value extracted and plotted onto a single plot to create the cyclic stress-strain curve.
Cyclic Hardening
This is a behavior exhibited by materials that, when subject to cyclic loading, actually strengthen with time. This is illustrated by the hysteresis loops becoming taller and skinnier on stress-strain space. The yield strength become greater.
Cyclic Softening
This is a behavior exhibited by materials that, when subject to cyclic loading, weaken with time. This is illustrated by the hysteresis loops becoming shorter and fatter on stress-strain space. The yield strength lessens
.
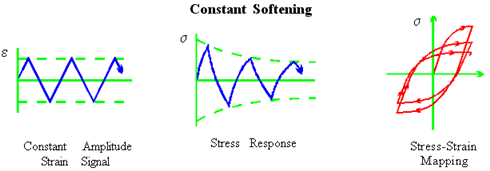
da/dN Curve
This is the crack growth rate (da/dN). It is a material characteristic and as such, is treated as a material data set and is obtained by experiments. It relates the growth rate of a crack to stress intensity (ΔK), or in other words, the driving force of the crack. There are three regions on a da/dN curve. Region 1 is the threshold region where the driving force is not great enough to grow a crack (like a fatigue limit). Region 2 is the linear region of the curve where behavior is described by the Paris equation. Region 3 is where static or fast fracture occurs as the driving force reaches or becomes very near to the fracture toughness of the material.
Damage (Log of Damage)
Damage is the reciprocal of Life. Sometimes it is reported in log base(10) units mainly for convenient contour plotting.
Damage Summation
This is the mechanism of summing the damage from the various stress cycles. All cycles are identified using rainflow cycle counting. Damage due to each is determined from the appropriate damage curve such as an S-N or strain-Life curve. Damage is then summed using the linear damage summation law as defined by Palmgen and Miner which simply states that each cycle causes a damage which is equal to 1/(number of cycles to failure at that load level). When you apply a series of cycles, damage is added up linearly until the total is unity (1) when failure is predicted. Fatigue damage is a non-linear process, but we find that if we have a fairly random repeated sequence it works satisfactorily.
Damage Tolerant
This is a fatigue life design philosophy which adopts the crack growth method and is used in conjunction with the fail safe philosophy. A crack or flaw is assumed to exist and its growth rate determined to set up specific inspection periods to ensure that the flaw will not grow to any critical size between these inspections.
Deterministic
This is a loading event which can be determined at any point in time, such as a constant amplitude sine wave. Repeatable loading falls into this category. This is in opposition to a random load where no events can be determined at any given point in time or more specifically, where the next sequence of events cannot be determined from any previous events.
Durability
Durability is the conglomeration of all aspects that effect the life of a product and usually concerns itself with much more than just fatigue and fracture, but also loading conditions, environmental concerns, material characterizations, and testing simulations to name a few. A true product durability program in an organization that takes all of these aspects and more into consideration.
Elastic
Elastic behavior refers to a component or material, which when subject to loading conditions that cause structural deformation, if removed, returns to its original state. No permanent state of deformation is left when the loads are removed. Linear elastic analysis denotes that as the loads vary, the responses vary in a linear and elastic manner relative to the loads. For instance if you double or triple the load, the responses will double or triple respectively. Whether the stresses exceed the yield or even the ultimate stress is not taken into account.
Elastic-plastic Correction
Endurance Limit
This is similar to the fatigue limit and is an imposed limit of reversals on strain-Life curves above which the component is said to have infinite life. This limit, referred to as the material cut-off, is set to 2e8 reversals but can be changed by the user.
Failure Criterion
The criterion that defines failure such as catastrophic failure into two or more pieces, until an engineering crack of 2mm appears, or until a crack reaches a critical size to be deemed unsafe. Understanding the failure criterion in a fatigue analysis is very important. The material properties used in any fatigue analysis, be it an S-N curve, strain-Life curve, or crack propagation da/dN curves, define the failure criterion.
Fail Safe
This is a fatigue life design philosophy which adopts the total life (S-N) method generally where failure cannot be tolerated. Therefore built in redundancy is generally used such that if a failure were to occur, the structure would fall into a state that it would survive until repair can be accommodated.
Fatigue
This is a failure under a repeated or otherwise varying load which never reaches a level sufficient to cause failure in a single application. The initiation and growth of a crack, or growth from a pre-existing defect, until it reaches a critical size, such as separation into two or more parts
Fatigue Concentration Factor, Kf
This is similar to the stress concentration, Kt, except it accounts for the fact that small notches have less effect on fatigue than is indicated by Kt. This has led to the idea of a fatigue concentration factor, Kf, which is normally less than Kt, being introduced and being used to replace Kt within Neuber’s rule. Kf is related to Kt according to
where p’ is a material constant dependent on grain size and strength and r is the notch root radius.
Fatigue Limit
This is a stress level below which no fatigue failures will occur. See
Stress-Life (S-N), 540.
Fourier Analysis
In simplistic terms, Fourier analysis is the ability to represent a finite length of time signal by the sum of a series of sine waves with varying amplitudes, frequencies, and phases.
Fracture
A fracture is the growth or propagation of a crack once it has been initiated. Fracture also denotes sudden breakage of a component or structure in two. However for the purposes of this manual it refers to the life prediction method of crack growth as implemented using LEFM. See
LEFM, 530.
Fracture Mechanics Triangle
The fracture mechanics triangle states that if any two of the three variables are known, through fracture mechanics and their relationships to one another, the other can be determined.
Frequency Domain
The time domain relates a variable (stress) to time and describes how the variable changes with time. Time signals can also be represented in the frequency domain which relates the variable to frequency, describing how that variable changes with or is affected by frequency. The time and frequency domains present the same information in different ways, helping the engineer understand the effect a signal or response may have on a structure. Consider, for example, a random signal which when converted to the frequency domain shows only content at 10 Hz. This signal when applied to a structure with natural frequencies well above 10 Hz may not be damaging at sufficiently low enough levels. However if the structure has natural frequencies in the 10 Hz range, the signal would be far more damaging. Being able to view a signal in the frequency domain can alert an engineer to this potential danger.
Gaussian
For a random signal and for most engineering purposes the amplitude Probability Density Function (PDF) will be approximately Gaussian. This means that the density distribution will take on a bell like curve as shown here where the highest levels of the signal are the least probable of occurring. See also
Power Spectral Density (PSD), 535.
Gerber Mean Stress
This is a mechanism to correct for a non-zero mean stress range for the S-N method. See
Mean Stress Correction, 531.
Goodman Mean Stress
This is a mechanism to correct for a non-zero mean stress for the S-N method. This is a more conservative method than the Berber one. See
Mean Stress Correction, 531.
High Cycle Fatigue (HCF)
This is the ability of a component or structure to withstand or survive many stress cycles. S-N analysis applications deal completely in the high cycle regime and are not valid in the low cycle regime. The technical definition of high cycle versus low cycle fatigue is where the elastic and plastic strain-Life curves cross each other on the strain-Life plot. This is known as the transition life above which is high cycle fatigue and below which is low cycle fatigue. It is clear that above the transition life elastic events dominate and below it, plastic events dominate. S-N analysis does not compensate for plastic events in an adequate way as the strain-life method does and for this reason is not a good choice for low cycle fatigue problems. The strain-life method can handle both high and low cycle fatigue problems. The transition life is generally around 104 or 105 cycles and is material dependent.
Hysteresis
This is a material behavior that is illustrated by loading a material beyond its yield point and then unloading it and perhaps reversing the load until it yields in compression and cycling. When the stress and strain are cross plotted, they create plots such as the one shown here. Each loop is a hysteresis loop. This phenomenon is know as the Baushinger effect after the German engineer that first documented this behavior of most metallic materials.
Inverse Fourier Transform
The ability or methodology of converting a frequency domain signal back into the time domain by recreating the time signal from a power spectral density (PSD) function is called the Inverse Fourier Transformation. Because no phase information is kept with a PSD, random phases are created. The regenerated time signal will not be exactly the same as the original but will be statistically equivalent.
Irregularity Factor
This is a parameter for describing or characterizing a process such as a time signal or a power spectral density function. For a time signal, it is defined as the ratio of the number of times a signal passes upward in a positive manner through the mean of a signal divided by the number of peaks. In the frequency domain, the irregularity factor is determined from its moments. The irregularity factor takes on values between zero and one, one describing a narrow band process and non-zero values describing wide or broad band processes. A value of unity describes a process whose peaks and adjacent valleys are roughly the same order of magnitude but of opposite sign whereas a value of near zero represents a signal that has an infinite number of peaks versus upward mean crossings, e.g., a dominate sine wave with noise superimposed on top of it.
K-Solution
LEFM
Linear Elastic Fracture Mechanics. This is the art of crack growth prediction as determined from linear elastic stresses. It assumes only a localized plastic zone around the crack tip and uses the stress intensity or driving force of the crack to determine crack growth rates according to the Paris equation.
Life (Log of Life)
The Life (Log of Life) is the result reported as to how long a component or structure will last. This life can be reported in terms of stress cycles or reversals survived, however this is usually not a convenient way of reporting it. Time series are generally given some sort of fatigue equivalent units such as laps, miles, hours, missions, etc., which are more descriptive to a user in describing the life. A repeat of a time history may have many stress cycles but can be described as representative of, say, 30 times around a cobblestone test track. The life is then reported as laps. Because the computed life of a component can vary dramatically from location to location on the component itself, the life is often reported in log (base 10) units. This is convenient because the spread can be from some small finite number (1000) to infinite life (the cutoff being around 1018). This helps spread out contour bands on graphical plots for better visualization and for xy plots.
Local Strain
Low Cycle Fatigue (LCF)
Low cycle fatigue is the inability of a component or structure to withstand or survive many stress cycles. See
High Cycle Fatigue (HCF), 528.
Material Cut-off
Material S-N
This is an S-N curve that relates local stress (σ) to life. These types of curves are generally obtained through material tests of highly polished test coupons where the monitored stress is the stress experienced at the failure location. These type of S-N curves are geometry independent; that is, the S-N curves are valid for any geometry and are only dependent on the actual material that they represent. All plasticity modeling is built into the curve.
Mean
Mean is the average value of a cycle or signal. It is the maximum plus the minimum divided by two for a simple constant amplitude oscillating signal as shown here. Note that the two small cycles in the stress-strain plot have the same strain range but have different mean stress.
Mean Stress Correction
This is a technique for correcting or compensating for non-zero mean signals when looking up damage on damage curves that have been created with zero mean (R=0) signals in a test laboratory. Various methods exist for both the S-N and strain-life methods. Fracture mechanics uses different da/dN curves for different R-ratios.
Miner’s Constant
Miner’s constant is the damage summation constant that defines failure, usually set to unity (1). See
Damage Summation, 523.
Monotonic Properties
Monotonic material properties are those that are obtained from a material test. Test coupons are placed in servo-hydraulic machines and loaded in a single application of steady load increase through the yield point of the material and to ultimate fracture of material. From these tests come various material parameters such as Young’s Modulus (E), the yield strength (σy), and the ultimate tensile (UTS). The load is not reversed, nor is it cycled to obtain these properties.
Morrow Mean Stress
A mechanism to correct for non-zero mean stress for the strain-life method. See
Mean Stress Correction, 531.
Multiaxial
Multiaxial means that the stress state is not uniaxial. More than one principal stress exists. The biaxiality ratio, ae, defined as the minimum in-plane stress divided by the maximum in-plane stress (for surface stresses), is non-zero. There are two different degrees of multiaxial stress states: proportional and non-proportional. Proportional multi-axial or proportional loading refers to the principal stresses always being in proportion to one another in magnitude and are stationary. Stationary means that the principal stress axes do not rotate significantly with time or in other words, the maximum and minimum principal stresses are always in the same direction. Non-proportional loading is the opposite of this where the two principal in-plane stresses are not proportional to each other at any given time, nor is the principal stress axis always in the same direction.
Narrow Band
This is a signal which contains frequency content predominantly at or around one frequency which when converted to the frequency domain appears as single peak spanning only a portion of the frequencies.
Neuber’s Rule
This is one of a few mechanisms to correct for plasticity given only elastic stresses and strains. The Neuber method enables us to predict elastic-plastic stress and strain by providing a way of estimating the amount of stress and strain redistribution. You should remember that this is an approximation! Basically the elastic strain excursion is calculated from the FE model, and the stress is assumed to be ε*E. Then the elastic-plastic stress and strain excursions is estimated by drawing a rectangular hyperbola through this point and seeing where it intersects the hysteresis curve.
Non-proportional Loading
Notch Correction
This is a term that is adopted in the FE-fatigue world to signify the correction from purely elastic stresses and strains to elastic-plastic stresses and strains. See
Elastic-plastic Correction, 524. Historically the term comes from determination of stress at a notch while taking measurements away from the notch and using a stress concentration factor, K
t, knowing that the material has yielded in the notch area and an additional correction needs to be made to determine the true stress (and/or strain).
Paris Equation
This is the main equation that governs the LEFM (crack growth) method and relates the crack growth rate (da/dN) to stress intensity (ΔK).

C and m are material constants.
Plasticity
Fatigue does not generally involve major changes to the properties of the bulk of the material in a component. In most components that have failed by fatigue, the processes that lead to the fatigue failure are confined to the region around the crack tip. Fatigue is always caused by plastic deformation. Plasticity is an irreversible process of deforming the material beyond its yield point. Some who have experienced fatigue failures may say “there is no plastic deformation in my component,” or “the FEA results showed that all stresses were below yield.” If there is a fatigue failure, then there must be plastic deformation, even if it is confined to only a few grains, or to a very small region around the tip of a crack or a notch.
Power Spectral Density (PSD)
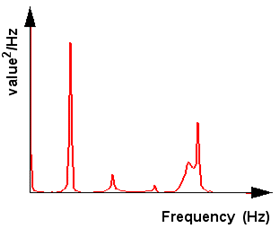
The term originated with electronic engineers in the 1940’s trying to characterize equipment noise. The PSD is a way of describing a random time signal. A random signal is random because there is no way of predicting a future section of the signal from previous sections. Therefore some sort of statistical method of describing these signals was devised. By taking a time signal, squaring it, and taking its average you get what is called the mean square value. If the squared signal is passed through a low pass filter at various cut-off frequencies, the mean value can be plotted as a function of frequency. The slope of this curve describes the density of the mean square with respect to frequency and is called a “spectrum.” The term “spectral density” comes from the fact that it is a property with respect to frequency such as a rainbow which is the variations of frequency in the colors of visible light. The term “power” dates back to the electrical engineers who used power as the key parameter. Dynamicists have simply adopted the term. In simple summary, a PSD is nothing other than an equivalent representation of a random time signal in a different domain, which has certain advantages over the time domain. In terms of Fourier analysis, the area under any infinitesimal strips of the PSD represents the mean square of the sine wave at that frequency where a time signal is made up of a number of sine waves summed together.
Probability Density Function (PDF)
Two important Probability Density Functions (PDF) can be computed from a stress or strain time history. These are the amplitude and peak PDFs as shown. The best way to visualize these parameters is to draw tram lines horizontally through the time history and then count either the number of times the signal crosses the tram lines or the number of times a peak occurs in-between the tram lines. The complete PDFs are obtained by repeating this process for all horizontal levels in the signal. For most engineering purposes the amplitude PDF will be approximately Gaussian. Furthermore, for a narrow band process the peak PDF will be approximately equivalent to the Rayleigh PDF. A PDF, therefore, is the probability of a certain stress or strain level occurring and is represented as a density distribution.
Proportional Loading
R-Ratio
This is a measure of the mean stress or the mean of constant amplitude signal or the mean of a stress cycle. R = -1 is a fully reversed signal or a cycle with zero mean. R = 0 is a signal which goes from zero to a maximum value and returns to zero. R = infinity is the reverse where the signal goes from zero to a negative maximum value and back to zero.
Rainflow Cycle Counting
Random Vibration
This is excitation due to loading which is random in nature. That is to say that at any particular point in time it is impossible to determine anything specific about the loading. It can only be described by its statistics such a mean level, rms, standard deviation, etc. This is in opposition to a loading event which can be determined at any point in time, such as a constant amplitude sine wave. Random vibration is usually dealt with in the frequency domain by converting signals to power spectral density functions (PSDs).
Range
Range is the total absolute magnitude between the maximum and minimum values of a cycle. Note that the two small cycles in the stress-strain plot have the same stress and strain range but have different mean stress.
Reference Location
When dealing with component S-N curves, this is the location on the test specimens used to create the S-N curve. The nominal stress axis of the S-N curve relates stress levels at this location to failure. When using a component S-N curve in conjunction with finite element models you must know the equivalent location (reference location) as only stress from this location relates to the S-N curve.
Regression Analysis
Regression analysis is the art of taking measured data such as that for an S-N curve and determining an equation to describe the curve from the raw data, also called curve fitting.
Residual Stress
This is a permanent stress that is left behind in a component or structure after unloading. Residual stress can be caused or induced in a number of ways such as shot peening, overloads, and manufacturing processes to name a few. Residual stresses can be tensile (positive) or compressive (negative) in nature and can be beneficial to bettering fatigue life (compressive) or detrimental (tensile).
Root Mean Square (rms)
By taking a time signal, squaring it, then taking the average, you get the mean square of the signal. If you take the square root of the mean square of the signal you get the root mean square (rms). The rms is a parameter that allows you to gauge the overall intensity of a signal relative to another random signal.
Safe Life
This is a fatigue design philosophy which adopts the crack initiation method. In general it is applied to relatively inexpensive components which can easily be thrown away and replaced. In addition it is applied to structures or parts where the initiation of a crack takes up the majority of the life relative to the growth of the crack or where it is intolerable to have a crack in the structure. This philosophy generally produces fairly optimized structures and is used heavily in the ground vehicle industry. A failure of a component designed with this philosophy should not have catastrophic consequences.
Sample Rate
When measuring a signal, the sample rate is the number of times you take a sample in a given period of time, usually one second. It is the frequency of samples in number/second. Sampling too slowly can cause important loading events to be missed.
Spectral Moments
Spectral moments are used to obtain other statistical properties of the PSD. The n-th spectral moment

of a PSD is defined by
Stress Concentration Factor, Kt
This is a factor which relates stress at one point in a structure to stress at another point. For example, the stress concentration factor for a large plate with a hole is three (3). This relates the nominal stress (P/A) at an area away from the hole to the stress at the radius of the hole. Concentration factors have come about due to the fact that it is difficult to place a measurement device directly on the highest stressed area. Therefore some factor had to be established to convert measured response to actual responses at critical locations. In FE fatigue based analysis, Kt is generally taken as unity (1), since in this case we do know the stress at the critical area. In fact we know the local stress at all locations.
Stress Intensity
In simplistic terms, this is the driving force that causes a crack to propagate forward. It controls the stress around a crack tip and is know as K (not to be confused with Kt or Kf, the stress and fatigue concentration factors). When the magnitude of K reaches the fracture toughness of a material, failure occurs. K is a function of the crack length, a, the nominal or far field stress away from the crack, and other geometric dimensions of the component or structure and has units of stress-length1/2.
Strain Hardening
Strain-Life (ε-N)
This is a fatigue life prediction method commonly referred to as “crack initiation,” or “local strain.” It only concerns itself with the initiation of a crack. The method is called “strain-life” because it relates local strain to life. It is a fairly recent and well accepted method of fatigue life prediction brought about by the work of many but principally the Americans, Manson and Coffin in the mid 1950s. This work would not have been possible without the invention of the servo-hydraulic test machine. These machines allowed strains to be precisely controlled as opposed to stresses which are near impossible to control. Because of this the scatter in material data for the strain-life method is much less than that of the S-N method and a more accurate prediction of fatigue life can be made.
Strain Softening
Stress-Life (S-N)
This is a fatigue life prediction method commonly referred to as “total life” because it does not make a distinction between initiating or propagating a crack but instead considers only the total life of the component until failure into two or more pieces. The method is called “stress-life” because it relates nominal or local stress to life. It was the first method of fatigue life prediction conceived by the German, August Woehler in the late 1800’s due to his work in the railway industry. His famous rotating-bending tests gave rise to the concept of the S-N curve. These curves are generally denoted in log units and some materials exhibit a “fatigue limit,” a stress level below which no fatigue failures will occur.
STW Mean Stress
This is a mechanism to correct for non-zero mean stress for the strain-life method. See
Mean Stress Correction, 531.
Surface Resolved Stresses
Surface resolved stresses are the stress on the surface of a structure or component which is said to be in a state of plane stress. The two principal stresses are in the plane of the surface while the third principal which is normal to the surface is zero. Finite element shell element models produce surface resolved stresses by default. However many solid element models produce stress results in elemental coordinate systems and need to be transformed into surface resolved stresses. Surface resolved stresses are needed to correctly calculate biaxiality ratios and perform multiaxial assessments
Total Life
Transfer Function
A Transfer Function is a way of relating one quantity to another. In the frequency domain the structure is modeled by a linear Transfer Function relating input loads to output responses. The output from the model is expressed as a PSD. In frequency response analysis these Transfer Functions are determined by subjecting the model at the input load point to a series of sine waves with unit amplitude over the frequency range of interest. Multiplying the input PSD of load by the Transfer Function then gives the response PSD.
Uniaxial
This is the stress state of a component or location in a component where only one principal stress exists, all others being zero. The biaxiality ratio, ae, defined as the minimum in-plane stress divided by the maximum in-plane stress (for surface stresses), is zero in this case. The principal stress is also stationary; that is, the principal stress is always in the same direction and not rotating such as a rod in tension.
White Noise
White noise is a signal which contains frequency content from all frequencies and when converted to the frequency domain, is a constant line. A sharp sudden impact is also a form of signal which contains content at all frequencies.
Wide Band
Wide band is a signal which contains frequency content at more than one frequency which when converted to the frequency domain can appear as multiple spikes or as a broad curve spanning multiple frequencies.